Six-Rayed Star Enstatite from Madagascar
- Details
- Created: Saturday, 13 June 2020 10:58
Initially published as a Gem note in the Journal Of Gemmology of the Gem-A. 2019, 36(8), 688–690.
http://doi.org/10.15506/JoG.2019.36.8.688
Enstatite may display asterism with four, six or eight rays, although it has been documented from only a few localities: southern India (Eppler 1967[1], 1971[2]), Ratnapura in Sri Lanka (Henn & Bank 1991[3]) and more recently Norway (Schmitz et al. 2016[4]). Enstatite is the Mg end member of the enstatite-ferrosilite series, the orthopyroxenes of the enstatite group. Historically, the series has been divided into intermediate members such as ‘bronzite’, hypersthene, etc. (Dunn 1975[5]; Deer et al. 1997[6]) according to Fe content. ‘Bronzite’ is not recognized by the International Mineralogical Association but occasionally it may be encountered in the literature.
Recently, star enstatite was found at a new locality: Madagascar. During the June 2019 Sainte-Marie-aux-Mines Mineral & Gem Show, the 1.24 ct cabochon in figure 1 was donated to the author for characterisation by Renan Deschard, a mining prospector in Madagascar.
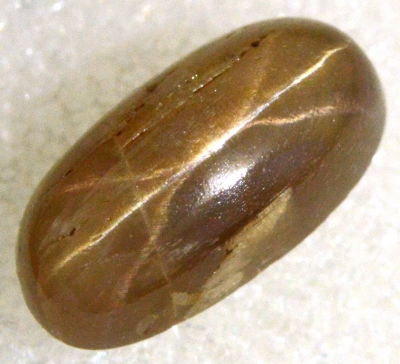
six-rayed asterism. The strongest rays are parallel to the length of
the stone. Some cleavage and parting are also visible. The cabo-
chon measures 9 × 5 × 3 mm.
Shape | oval cabochon |
Size | 9 x 5 x 3 mm |
Color | light brown |
Lustre | vitreous |
Weight | 1.24 |
SG | 3.25 |
RI | 1.660 - 1.670 |
DR | 0.010 - Biaxial positive |
Pleochroism | strong, in orangey brown, greyish green and grey |
Polariscope / Conoscope | just translucent, not applicable |
SWUV | inert |
LWUV | inert |
Magnetic susceptibility N52 | slightly attracted to an N52 (neodymium) magnet |
Chelsea filter | inert |
Handheld spectrocope | continuous absorption in the violet and blue regions with two sharp bands in the green around 505 and 545 nm |
Table 1. Observational and measured properties. These data are consistent with those reported for enstatite by O’Donoghue (2006[7]).
Light scattering from a dense network of inclusions formed the six-rayed star, with the strongest rays seen parallel to the length of the cabochon. Microscopic examination (figure 2) revealed parallel acicular (needle-like) inclusions (up to 2 µm wide and 150 µm long) aligned in three directions and intersecting at approximately 57° and 62°. The intersection angles are consistent with the orthorhombic pseudo-hexagonal symmetry of enstatite and published data: 57° and 64° (Eppler 1967[1], 1971[2]); 57° and 61° (Henn & Bank 1991[3]); and 64° (Schmitz et al. 2016[4]). Eppler (1967[1]) suggested rutile as a possible inclusion; Henn & Bank (1991[3]) ascribed the asterism to hollow channels; Gübelin & Koivula (2008[8]) indicated rutile or sillimanite in Sri Lankan enstatite; and Schmitz et al. (2016[4]) identified ilmenite in Norwegian enstatite. Further investigation such as micro-Raman spectroscopy would be necessary to identify the inclusions in the Madagascar enstatite.
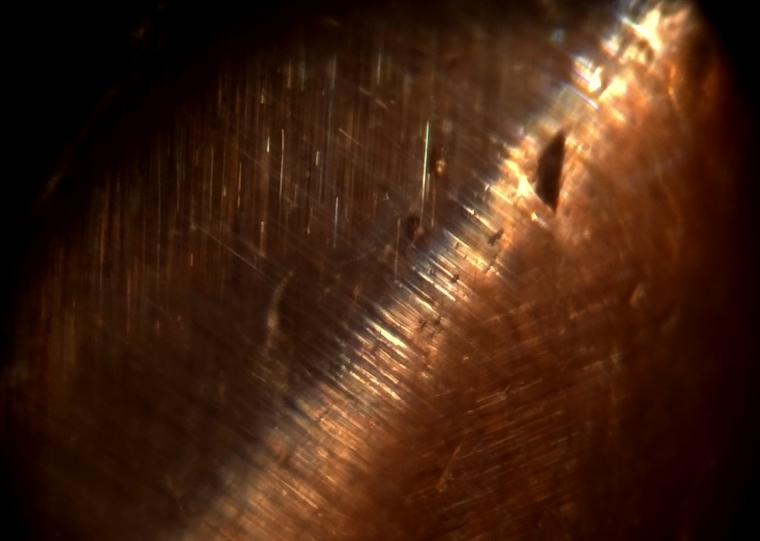
57° and 62°. Image width 1.0 mm.
Infrared reflectance spectroscopy:
Infrared reflectance spectra were collected from several spots on the cabochon, and all yielded the same pattern without any distinct difference (figure 3). The spectrum is characteristic of enstatite (see the Enstatite-Ferrosilite database; Chihara et al. 2002[9]).
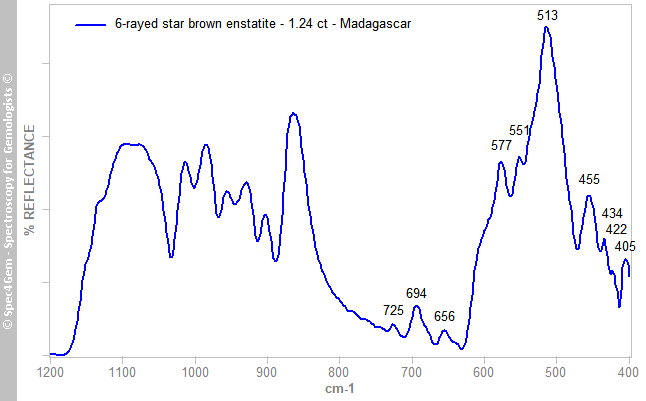
UV-VIS-NIR spectroscopy:
Vis-NIR spectra (figure 4) were collected with the beam entering from the top and oriented nearly perpendicular to the base of the cabochon. Due to the beam’s orientation, only two spectra were acquired: one for the orangey brown colour and one for the greyish green colour. The spectra were consistent with reference spectra for enstatite (see, e.g., the Enstatite-Ferrosilite database; Caltech[10]).
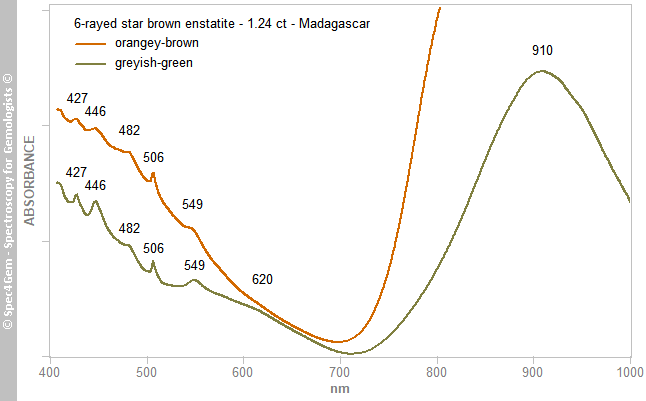
Across the visible range, both spectra showed a continuous absorption increasing towards the UV region (weaker for the greyish green direction), with a superimposed series of narrow bands at 427, 446, 482, 506 and 549 nm; the 506 nm band is characteristic of enstatite.In the near-IR range, both spectra show a broad, strong absorption band centred at 910 nm (stronger in the orangey brown direction). The continuous absorption in the visible range coupled with the near-IR band creates a transmission window in the orange and red region, which is narrower in the orangey brown spectrum than in the greyish green one. The narrower transmission window produced the orangey brown colouration, while the broader one resulted in the greyish green colouration since more green was transmitted. The continuum towards the UV and the strong band in the NIR are related to Fe2+ substituting for Mg2+ in the M1/M2 sites, and the narrow bands between 400 and 600 nm are Fe2+ spin-forbidden absorption bands (Hazen et al. 1978[11]; Zhao et al. 1986[12]). Thus, the stone is coloured by Fe2+.
Additionnal discussion about enstatite polymorphism (unpublished in the Gem note)
Enstatite (also referred as orthoenstatite, the orthorhombic form) has few polymorphs among which clinoenstatite is the monoclinic form. The clinoenstatite's RI, birefringence, optic sign and SG are similar in all respects to those of the enstatite (Deer et al. 1997, pp. 118–119[6]), however the characteristic pale-pink to green pleochroism distinguishes many (ortho-)pyroxenes from clino-pyroxenes. The strong orangey brown to greyish green of this sample is consistent even if not exactly similar to that pleochroism, it points enstatite out.
The enstatite polymorphism has been studied with IR and Raman spectroscopy, Chihara et al. (2002)[9] indicated that far-infrared (~400–10 cm−1) spectral features provide the best way to distinguish them, however it is also possible to use the mid-infrared range (~4000–400 cm−1). The (ortho-)enstatite and the clinoenstatite mid-IR spectra from that study are provided in figure 5 for comparison to the sample's one. They were numerised and the frequency scale converted to a linear one, therefore they show some slight discrepencies with the sample in the bands position that are slightly shifted. The discriminating features pertain to the variation of the bands at 725, 694, 484, 455, 434, 422 and 405 cm–1 shown in the pink highlighted regions of figure 5. This sample of enstatite from Madagascar shows an IR spectrum which is closer to the enstatite's one than to clinoenstatite's one.
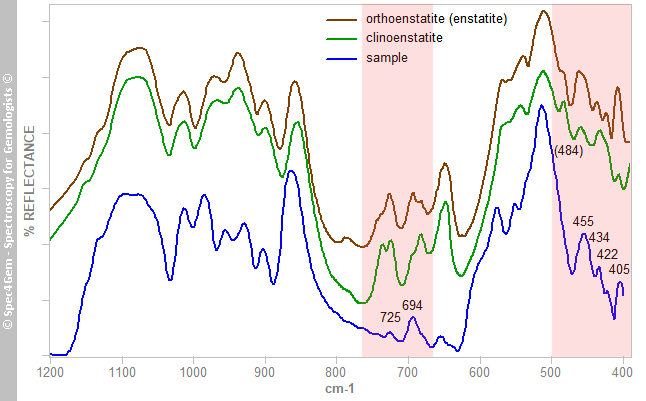
[1] Eppler, W.F. 1967. Star-diopside and star-enstatite. Journal of Gemmology, 10(6), 185–188, http://doi.org/10.15506/JoG.1967.10.6.185.
[2] Eppler, W.F. 1971. Some rare materials. Journal of Gemmology, 12(7), 256–262, http://doi.org/10.15506/JoG.1971.12.7.256.
[3] Henn, U. & Bank, H. 1991. Sternbronzit aus Sri Lanka. Zeitschrift der Deutschen Gemmologischen Gesellschaft, 40(2–3), 145–148.
[4] Schmitz, F., Stephan, T. & Müller, S. 2016. Gem Notes: Polymer-filled star enstatite from Norway. Journal of Gemmology, 35(2), 96–118, http://doi.org/10.15506/JoG.2016.35.2.96.
[5] Dunn, P.J. 1975. On gem orthopyroxenes: Enstatite and bronzite. Gems & Gemology, 15(4), 118–122.
[6] Deer, W.A., Howie, R.A. & Zussman, J. 1997. Rock-Forming Minerals: Single-Chain Silicates, 2nd edn. The Geological Society Publishing House, Bath, 680 pp.
[7] O’Donoghue, M. (ed) 2006. Gems, 6th edn. Butterworth-Heinemann, Oxford, 873 pp.
[8] Gübelin, E.J. & Koivula, J.I. 2008. Photoatlas of Inclusions in Gemstones, Vol. 3. Opinio Publishers, Basel, Switzerland, 672 pp.
[9] Chihara, H., Koike, C., Tsuchiyama, A., Tachibana, S. & Sakamoto, D. 2002. Compositional dependence of infrared absorption spectra of crystalline silicates. Astronomy & Astrophysics, 391(1), 267–273, http://doi.org/10.1051/0004-6361:20020791.
[10] Pyroxene Visible Spectra - List of Visible Data Files on the Caltech Mineral Spectroscopy Server
[11] Hazen, R.M., Bell, P.M. & Mao, H.K. 1978. Effects of compositional variation on absorption spectra of lunar pyroxenes. 9th Lunar and Planetary Science Conference, Houston, Texas, USA, 13–17 March, 2919–2934.
[12] Zhao, S.-B., Wang, H.-S., Zhou, K.-W. & Xiao, T.-B. 1986. The spin-forbidden absorption spectrum of Fe2+ in orthopyroxene. Physics and Chemistry of Minerals, 13(2), 96–101, http://doi.org/10.1007/bf00311899.